Planktonic:
In-water Prevention Strategy
Substantial Supporting Field Data
Benthic:
In-water Prevention Strategy
Limited Supporting Field Data
Geoengineering involves the addition of phosphorus-binding (P-binding) elements to lake-bottom sediments to bind sediment phosphorus and control the release of phosphorus from sediment during low-oxygen conditions (for example, internal phosphorus loading control). Minerals containing aluminum have been employed for decades and are naturally abundant in the environment; aluminum content of soils and sediment is generally between 1% and 10% (Sorenson et al. 1974, USGS 1984). Natural lake water concentrations of aluminum range from 10 µg/L to100 µg/L (Wetzel 2001). Aluminum acts by converting iron-bound and pore water phosphorus to aluminum-bound phosphorus. Aluminum-bound phosphorus is stable under low-oxygen conditions and a wide pH range and is thus considered permanently inactivated after treatment. Aluminum is commonly applied as alum, sodium aluminate, and/or polyaluminum chloride (alum and sodium aluminate are applied together as a buffer in low-alkalinity waters). The material is typically applied as a liquid (by a treatment barge) to the lake surface, where it forms a floc that then settles to the bottom and is incorporated into the sediment. In some cases, a solid aluminum salt is applied. It is one of the few strategies used for internal phosphorus control that has extensive field evidence, and it is the only geoengineering material that has extensive field data for longevity, effectiveness, and safety.
Several factors determine the effectiveness and longevity of aluminum treatment. For example, aluminum treatment will be more effective for water bodies with significant internal phosphorus loading relative to watershed phosphorus loads. A high water-surface to tributary watershed area ratio increases treatment longevity, as a larger ratio is generally indicative of a lower flushing rate. An approximate cutoff is a 7.2 ratio of watershed to water body area. See Huser et al. (2016) for factors that may affect treatment longevity. Accurate aluminum dosing also increases effectiveness and longevity (Huser and Pilgrim 2014, Pilgrim, Huser, and Brezonik 2007). Longevity and effectiveness are greater for water bodies with stable thermocline and infrequent mixing. However, this does not preclude the use of aluminum for systems that mix, given that phosphorus transport from the lake bottom is mediated by mixing. Aluminum may be very effective for systems that mix, but longevity of a treatment is largely determined by new phosphorus inputs. Shallow systems experience more phosphorus load per unit lake volume compared to a deep lake, given equal watershed inputs. Although longevity is often reduced for shallow systems, primarily due to continued excess external loading of phosphorus, treatment can be used effectively for shallow lakes if factors such as benthic feeding fish, invasive aquatic plants, and best management practices (filtration for phosphorus removal) are also implemented (Bartodziej, Blood, and Pilgrim 2017). Trophic state is not a determinant of use, and aluminum can be used for a wide range of sediment phosphorus concentrations. For eutrophic systems, application is most often conducted in the spring and fall to avoid algal blooms or aquatic plants interfering with floc formation and settling. It is also notable that treatment effectiveness has been recently demonstrated for estuarine waters using polyaluminum chloride (Rydin et al. 2017).
PLANKTONIC AND BENTHIC |
EFFECTIVENESS
|
NATURE OF HCB
|
ADVANTAGES
|
LIMITATIONS
|
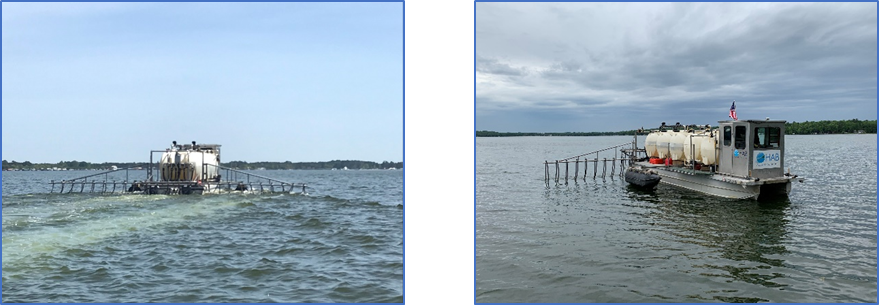
Figure 1. Applying P-binding compounds to a lake.
Source: J. Holz, HAB Aquatic Solutions.
Alternative methods include other P-binding material additions, such as modified bentonite clay (Robb et al. 2003), lime (Ca[OH]2, CaCO3), and ferric chloride (FeCl3) (Chorus and Bartram 1999). Note, however, that Triest, Stiers, and Van Onsem (2016) cautioned the use of lime, as lime-induced increases in lake pH (>8.0) select for cyanobacteria. Implementation of other strategies in the watershed can reduce incoming phosphorus (see Section 7 in ITRC 2021), thereby making in-water P-binding additions more effective and reducing need for reapplication.
COST ANALYSIS
Overall, aluminum treatment is considered to be cost-effective compared to other phosphorus control methods (Bartodziej, Blood, and Pilgrim 2017). The cost to apply aluminum by commercial applicators is typically quoted as per gallon applied. The cost per gallon applied is the market cost of the liquid aluminum product delivered to the site, plus a markup by the contractor to apply the product. The amount of aluminum needed is often determined by an aerial dose quoted as grams of aluminum per square meter of lake bottom area. This aerial dose is determined by the amount of phosphorus in the lake-bottom sediment. More phosphorus (often called mobile phosphorus) in the lake-bottom sediments requires more aluminum. The initial application is expensive, but aluminum treatments can be expected to last 11 years, resulting in a low average cost per growing season. The cost will need to be determined on a site-specific basis. Previous estimates from 14 studies indicate an average cost of $5,275/acre in U.S. dollars (see Appendix C.2 in ITRC 2021).
Relative cost per growing season: P-binding compounds
ITEM | RELATIVE COST PER GROWING SEASON |
Material | $$ |
Personal Protective Equipment | $ |
Equipment | $$$ |
Machinery | $$ |
Tools | $ |
Labor | $$ |
O&M Costs | $ |
OVERALL | $–$$ |
REGULATORY AND POLICY CONSIDERATIONS
You can expect a large range of comfort with this prevention approach, and each state will have a different aluminum treatment policy. The approval process may not be straightforward, as aluminum treatment does not fall under the jurisdiction of most established permitting programs. You will need to contact the state limnologist or appropriate permitting staff.
The primary regulatory hurdle with this method is the potential for aluminum-induced, short-term aquatic toxicity during application. Long-term effects (chronic toxicity) have not been observed for typically applied doses (Clearwater, Hickey, and Thompson 2014). The literature regarding aluminum aquatic toxicity is extensive and largely resulting from acid rain research in the 1970s and 1980s (see USEPA 2018 for an extensive review of aluminum toxicity). However, conditions in eutrophic lakes are very different compared to acidified lakes, considering the predominant condition where aluminum is acutely toxic (pH<5.5). The pH range in eutrophic lakes is most often in the neutral range (pH 7.0–9.0), and there is a large body of evidence demonstrating safe application of aluminum for phosphorus treatment, even in low-alkalinity waters. Numerous studies have shown that aluminum treatments had no adverse effect on aquatic life. In some cases, fish or benthic invertebrate abundances increased (Buergel and Soltero 1983, Glilman 2006, Narf 1985, Narf 1990, Smeltzer 1990). In contrast to acidification-related aluminum studies, water column aluminum concentrations have been shown to decrease in the water after the floc has settled to the sediment (Pilgrim and Huser unpublished). This is due to the reduction of particulate matter in the water (algae) that can bind with natural aluminum entering the water body. The increasingly common use of buffered alum and sodium aluminate treatment has improved the ability to regulate pH during treatment, and application during spring and fall has avoided potential complications with phytoplankton and cyanobacterial blooms.
CASE STUDY EXAMPLE
Step 1. Identify the depth of elevated mobile phosphorus in sediment (g mobile P per square m by cm depth).
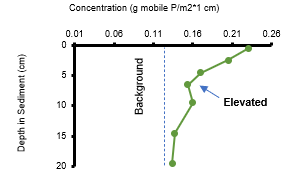
Step 2. Calculate the average concentration of mobile phosphorus in the sediment with elevated phosphorus.
Step 3. Identify the aluminum dose necessary to reduce mobile phosphorus to the desired level. This may reduce mobile phosphorus as much as possible or reduce it to background levels identified by sediment cores. Use the methods of Pilgrim, Huser, and Brezonik (2007) to identify the expected mobile phosphorus reduction. An example of this relationship is shown below.
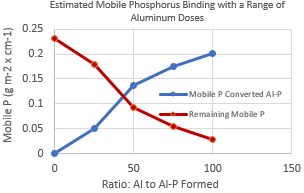
Step 4. Identify the depth of sediment to be treated and calculate the total dose to be applied to the lake as g aluminum/m2 of lake-bottom sediment (Huser and Pilgrim 2014, Pilgrim, Huser, and Brezonik 2007).
REFERENCES
Bartodziej, W. M., S. L. Blood, and Keith Pilgrim. 2017. “Aquatic plant harvesting: An economical phosphorus removal tool in an urban shallow lake.” Journal of Aquatic Plant Management 55:26-34.
Buergel, Pamela M., and Raymond A. Soltero. 1983. “The distribution and accumulation of aluminum in rainbow trout following a whole-lake alum treatment.” Journal of Freshwater Ecology 2 (1):37-44. doi: https://doi.org/10.1080/02705060.1983.9664574.
Chorus, Ingrid, and Jamie Bartram. 1999. “Toxic cyanobacteria in water: a guide to their public health consequences, monitoring and management.” Geneva: World Health Organization. http://www.toxicologia.org.ar/wp-content/uploads/2016/03/toxcyanobacteria.pdf.
Clearwater, Susan, Christopher Hickey, and Karen Thompson. 2014. “The effect of chronic exposure to phosphorus-inactivation agents on freshwater biota.” Hydrobiologia 728. doi: https://doi.org/10.1007/s10750-014-1805-9.
Glilman, Bruce. 2006. “Pre- and Post-Alum Treatment Survey of Honeoye Lake Macrobenthos: Comprehensive Field Inventory and Data Summary July 2005 and November 2006.” Department of Environmental Conservation and Horticulture, Finger Lakes Community College. https://hvaweb.org/Resources/Documents/Pre%20and%20Post-alum%20Survey%20of%20Honeoye%20Lake%20final.pdf.
Huser, B. J., S. Egemose, H. Harper, M. Hupfer, H. Jensen, K. M. Pilgrim, K. Reitzel, E. Rydin, and M. Futter. 2016. “Longevity and effectiveness of aluminum addition to reduce sediment phosphorus release and restore lake water quality.” Water Research 97:122-32. doi: https://doi.org/10.1016/j.watres.2015.06.051.
Huser, B. J., and K. M. Pilgrim. 2014. “A simple model for predicting aluminum bound phosphorus formation and internal loading reduction in lakes after aluminum addition to lake sediment.” Water Research 53:378-85. doi: https://doi.org/10.1016/j.watres.2014.01.062.
ITRC. 2021. “Strategies for Preventing and Managing Harmful Cyanobacterial Blooms (HCBs).” Washington, D.C.: Interstate Technology and Regulatory Council. Strategies for Preventing and Managing Harmful Cyanobacterial Blooms Team. https://hcb-1.itrcweb.org/.
Narf, R. P. 1985. “Impact of Phosphorus Reduction via Metalimnetic Alum Injection in Bullhead Lake, Wisconsin.” Madison, WI. https://dnr.wi.gov/files/PDF/pubs/ss/SS0153.pdf.
Narf, Richard P. 1990. “Interactions of Chironomidae and Chaoboridae (Diptera) with aluminum sulfate treated lake sediments.” Lake and Reservoir Management 6 (1):33-42. doi: https://doi.org/10.1080/07438149009354693.
Pilgrim, K. M., and B. J. Huser. unpublished. “Unpublished data from Minnesota and Sweden.”
Pilgrim, K. M., B. J. Huser, and P. L. Brezonik. 2007. “A method for comparative evaluation of whole-lake and inflow alum treatment.” Water Research 41 (6):1215-24. doi: https://doi.org/10.1016/j.watres.2006.12.025.
Robb, Malcolm, Bruce Greenop, Zoë Goss, Grant Douglas, and John Adeney. 2003. “Application of Phoslock™, an innovative phosphorus binding clay, to two western Australian waterways: preliminary findings.” Hydrobiologia 494. doi: https://doi.org/10.1023/A:1025478618611.
Rydin, E., L. Kumblad, F. Wulff, and P. Larsson. 2017. “Remediation of a eutrophic bay in the Baltic Sea.” Environmental Science and Technolology 51 (8):4559-4566. doi: https://doi.org/10.1021/acs.est.6b06187.
Smeltzer, Eric. 1990. “A successful alum/aluminate treatment of Lake Morey, Vermont.” Lake and Reservoir Management 6 (1):9-19. doi: https://doi.org/10.1080/07438149009354691.
Sorenson, J. R., I. R. Campbell, L. B. Tepper, and R. D. Lingg. 1974. “Aluminum in the environment and human health.” Environmental Health Perspectives 8:3-95. doi: https://doi.org/10.1289/ehp.7483.
Triest, Ludwig, Iris Stiers, and Stijn Van Onsem. 2016. “Biomanipulation as a nature-based solution to reduce cyanobacterial blooms.” Aquatic Ecology 50 (3):461-483. doi: https://doi.org/10.1007/s10452-015-9548-x.
USEPA. 2018. “Final Aquatic Life Ambient Water Quality Criteria for Aluminum 2018 EPA 822-R-18-001.” Washington, D. C.: U.S. Environmental Protection Agency, Office of Water. https://www.epa.gov/sites/production/files/2018-12/documents/aluminum-final-national-recommended-awqc.pdf.
USGS. 1984. “Element Concentrations in Soils and Other Surficial Materials of the Conterminous United States, Geological Survey Professional Paper 1270.” Alexandria, VA: U.S. Geological Survey. https://pubs.usgs.gov/pp/1270/.
Wetzel, Robert G. 2001. Limnology: Lake and River Ecosystems. San Diego, CA: Academic Press.